Mechanisms of epilepsy collapse onto parvalbumin interneuron dysfunction
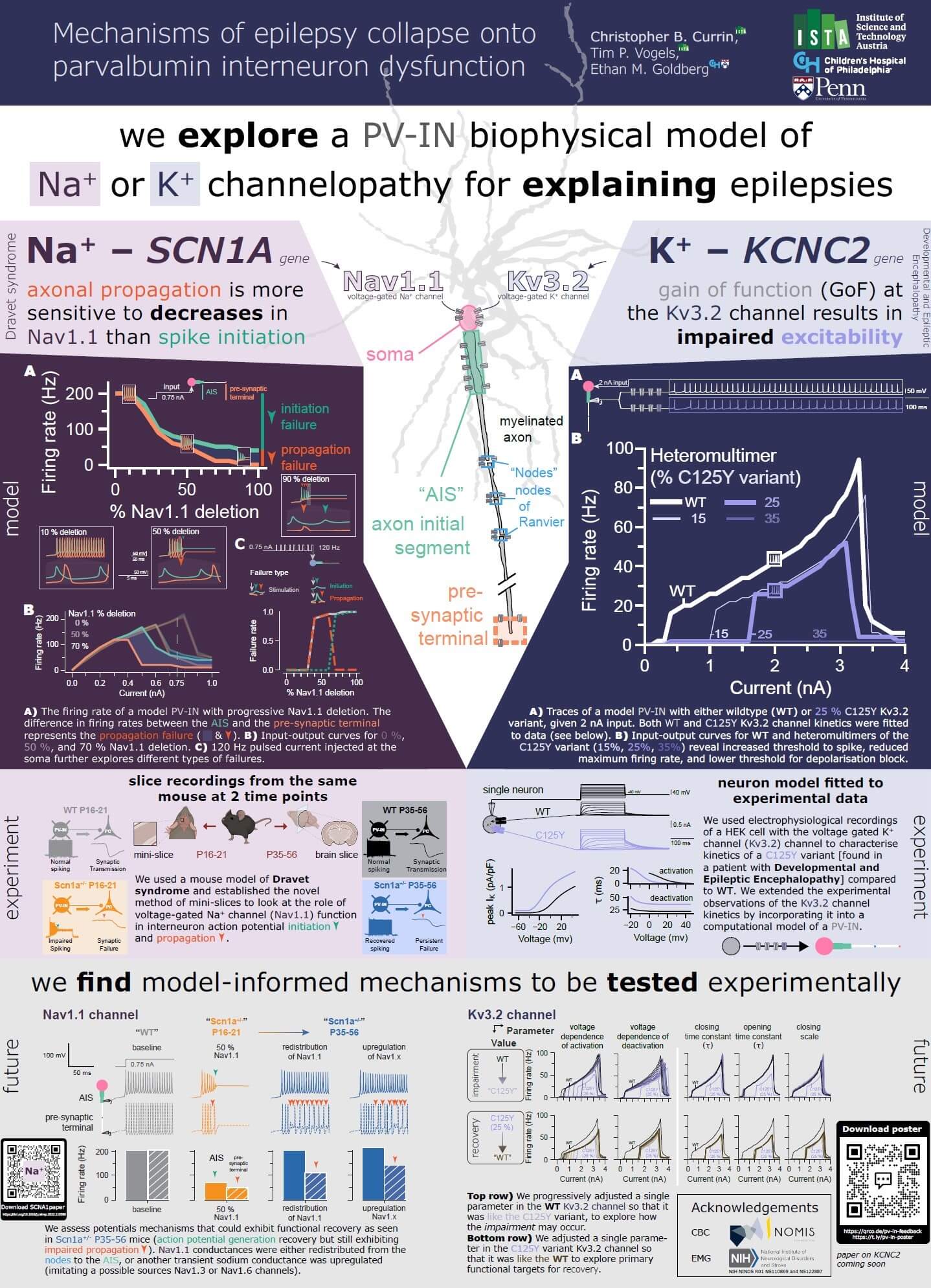
Christopher Brian Currin 1, Tim P. Vogels 1, Ethan M. Goldberg 2,3
- Institue of Science and Technology Austria, Am Campus 1, Klosterneburg, Austria
- Division of Neurology, Department of Pediatrics, The Children’s Hospital of Philadelphia, Abramson Research Center, Philadelphia, PA 19104, USA
- Department of Neurology, The University of Pennsylvania Perelman School of Medicine, Philadelphia, PA 19104, USA
Abstract
Ion channels dynamics have increasingly been implicated in the manifestation and maintenance of seizures. The majority of investigations focus on ion channel dynamics in pyramidal neurons, which are more commonly expressed than parvalbumin-positive (PV) interneurons in the cortex and hippocampus. PV interneurons, however, play a critical role in suppressing or preventing seizures by providing inhibitory restraint via fast action potential generation and propagation, in turn synapsing onto pyramidal neurons and typically hyperpolarising the post-synaptic membrane potential. Our research focused on sodium (Na+) and potassium (K+) channel dysfunction in experimental recordings and computational models that revealed two different mechanisms of impairment in interneurons that can lead to epileptic seizures.
First, we used a mouse model of Dravet syndrome and established the novel method of mini-slices to look at the role of voltage-gated Na+ channel (Nav1.1) function in interneuron action potential initiation and propagation. By looking at fine-grain electrophysiological recordings from the same mouse at different time points using the novel technique of “mini-slices”, the impairment of action potential initiation seen at early developmental stages was recovered by adulthood, but propagation was not. Using a computational model of a morphologically detailed PV interneuron and fitted to the experimental data, the mechanism of recovering action potential initiation but not propagation was suggested to be either via redistribution of Nav1.1 channels to the axon initial segment (AIS) or via upregulation of other sodium channels in the AIS.
Second, we explored a gain in ion channel function for a variant (C125Y) of the voltage gated K+ channel (Kv3.2) encoded by the KCNC2 gene. We used electrophysiological recordings of a HEK cell with the Kv3.2 channel to characterise kinetics of the C125Y variant compared to WT. We extended the experimental observations of the Kv3.2 channel kinetics by incorporating it into a computational model of a PV interneuron. We saw a substantial loss of neuronal output for a heteromultimer of the Kv3.2 C125Y variant (25 %), and investigated which were the most crucial parameters for pathological activity and potential therapeutic targeting.
Together, we performed both experimental and computational investigations into two novel mechanisms of epilepsy and seizures that both centred on PV interneuron dysfunction. These results have furthered our current understanding of the loss of inhibitory restraint in a network, and inform future directions of research using computational model-guided experiments.